What we must do to save science
ASU professor argues that scientists shouldn't simply create more knowledge, but address important, real-world problems
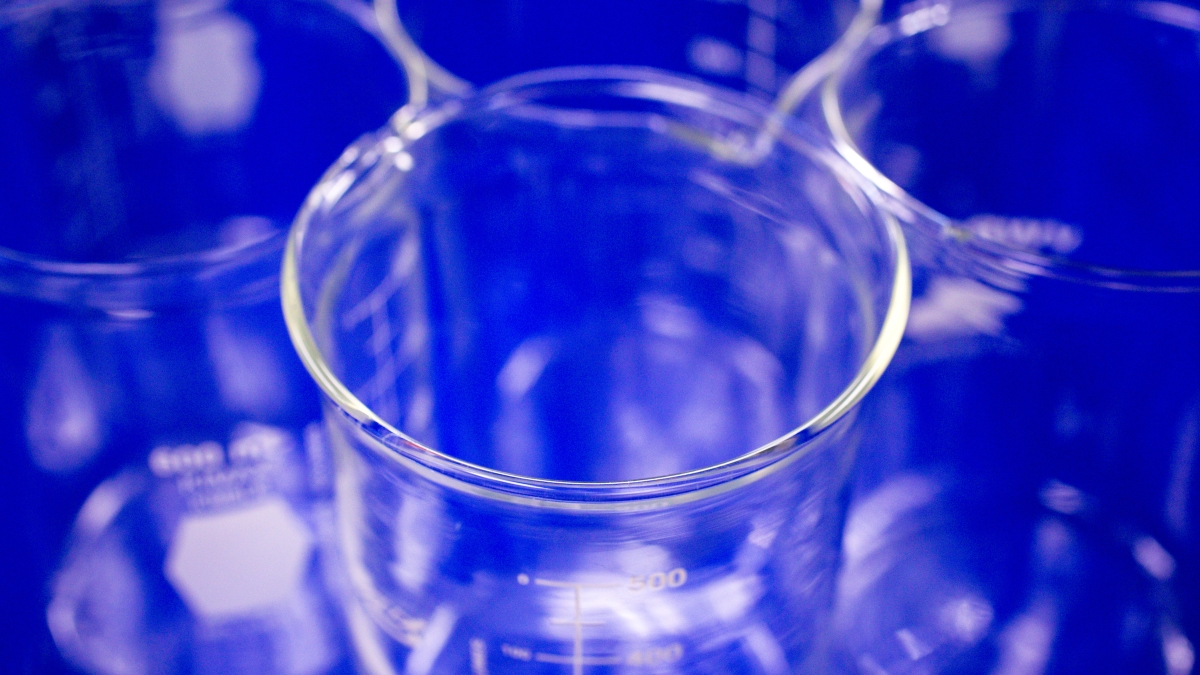
“Science, pride of modernity, our one source of objective knowledge, is in deep trouble.”
So begins “Saving Science,” an essay for The New Atlantis by Daniel Sarewitz, professor at Arizona State University’s School for the Future of Innovation in Society and co-director of the Consortium for Science, Policy & Outcomes.
Scientists are more productive than ever, turning out millions of articles covering an ever-expanding array of fields. But much of it, Sarewitz says, is turning out to be unreliable or flat-out wrong.
“Curiosity-driven” basic research that has long characterized science is fundamentally misguided, Sarewitz argues, and it has led to a crisis that we are only beginning to acknowledge.
Science must better engage with real-world problems, he says: Only by addressing important challenges — safe drinking water, disease treatments, better nutrition and more equitable economic prosperity, for example — can science fulfill its tremendous potential for social benefit.
Here, Sarewitz discusses the current state of the scientific enterprise, the influence ASU has had on his thinking, and how to begin resolving the problems he highlights.
ASU professor Daniel Sarewitz, who is co-director of the Consortium for Science, Policy & Outcomes, says we must be willing to talk about what works and what doesn’t in order for science to fulfill its potential for social benefit. Photo courtesy of Rathenau Institute, Netherlands
Question: Can you summarize the central argument of the essay?
Answer: I think there’s a broad awareness that the American science system, and science in general, is suffering from a range of challenges and stresses. The quality of science is being questioned; politics and science are increasingly hard to separate; many are dissatisfied with the rate of progress on big problems like cancer and climate change.
I wanted to show that the difficulties afflicting the science system are interrelated — and that they can’t be cured just by pouring more money into science! (But they could be made worse.)
Q: Can you describe the steps that have led to this moment of crisis in science?
A: Well I wouldn’t call them “steps,” so much as denial. As I write in the article, some of the important conditions that underlie the crisis were recognized 40 or 50 years ago, as with Derek Price’s recognition in the 1960s that the research enterprise was growing at an unsustainable rate, and Alvin Weinberg’s insight in the ’70s that science and politics were getting mixed together. So really what’s happening is at least in part a culmination of processes and stresses that have been building for decades.
Q: You call the idea that scientific progress is the result of “the free play of free intellects” a “bald-faced but beautiful lie.” This implies deception on the part of the postwar architects of American science. Why “lie,” rather than, for instance, “myth”?
A: Yeah, I thought long and hard about whether to use that word and decided, in the end, that it was justified. “The free play of free intellects” is a line from Vannevar Bush’s 1945 report “Science, The Endless Frontier,” which he wrote in order to secure federal support for the postwar scientific enterprise.
To keep funding flowing to all the scientists who’d been engaged in the war effort, he argued that technological progress begins with basic research (or so-called “pure” science) and proceeds linearly through development, application, and finally innovation. He knew, as an inventor and engineer, that this linear model of innovation was mostly wrong: innovations emerge through complex and continual interaction between science and technology.
But scientific leaders since Bush, not surprisingly, have typically been happy to pay attention only to the linear model, despite that fact that for many decades, studies of science and innovation have shown that directions of scientific advance — even for very basic science — are typically strongly influenced by and linked to technological advance.
Q: What kinds of science are we talking about? “Science” comprises a tremendous range of disciplines and institutions and activities. Does your argument apply to all of them?
A: This is a great question, and in fact the whole idea that there is one thing called “science” probably does more harm than good, since inevitably it makes people think that all sciences should aspire to being like classical physics — reductionist, quantitatively precise, and predictive. But many fields make their most important contributions without being any of those things (or at least not dominantly).
My essay mostly pertains to sciences that are trying to unravel complex phenomena, often with a social component (though not necessarily). This may include fields ranging from physical sciences like climate science, to life sciences trying to make progress on cancer, to social sciences trying to find a way to improve public education. But I’m not really talking much about sciences like cosmology, say, or subatomic particle physics, which no one expects to have a practical application — and where it really doesn’t matter if the results are true or not.
Q: What’s a current example of science working as it should? Are there fields that, in your view, are effectively solving important problems?
A: It’s less about fields than about how science is being organized. To me the most important part of the essay are the examples of how really smart and committed people are organizing science in different ways, to make it more accountable for achieving the goals that people are expecting and hoping for.
I very intentionally offered three really different examples — breast cancer research, environmental protection, and brain research — to show how the quality of scientific research, and its social value, are actually strongly connected through the way that science is organized, and the way accountability is understood and imposed.
Q: How do we figure out which social goals science should be working toward? Reorienting science to solve problems rather than produce more knowledge sounds effective, but debate over which problems science ought to be directed toward can be extremely contentious.
A: I don’t think this is a problem at all; the social goals for science are widely shared across society — and many of them were articulated in “Science, The Endless Frontier.” We want science to help improve our health, to help keep our environment clean, to provide jobs and economic growth and national security.
The problem is not the goals; it’s the organization of science to contribute to those goals that we need to be concerned about. As I write in the essay, the United States did a fantastic job organizing science to fight the Cold War, and this led to many other benefits for society that had little to do with defense, at least not directly. But we haven’t learned those lessons; in fact, we’ve decided to forget them.
Q: Has your work at Arizona State University influenced your thinking on this issue?
A: Of course. I came to ASU because of its amazing culture of collaboration and connectedness to the real world. And the mission of combining inclusion and service with cutting-edge, top-level research is exactly the way we need to think about organizing science — this is where science’s value to society is going to come from.
I think one thing we’re finding out with the current crisis is that the elite, ivory-tower model for science has created an inward-looking enterprise whose social value is increasingly questionable. ASU is inspiring to me because it has understood that being part of society and doing great work are synergistic.
Q: How does this play out in the future? There are trends in many fields, such as “precision medicine” or “big data,” that have led people to proclaim that we’re very close to transformational breakthroughs in our understanding and treatment of, for example, certain diseases. Is this just hype?
A: There is certainly hype, and lots of it. But there is also promise in equal measure. I’ve gone on for too long, but I’ll take the liberty of lifting a couple sentences from the essay: “In the future, the most valuable science institutions will be closely linked to the communities and contexts whose urgent problems need to be solved; they will cultivate strong lines of accountability to those for whom solutions are important; they will incentivize scientists to search for solutions, often technological ones, rather than to simply produce more knowledge. If we can organize more of science with these basic principles in mind, then I think we can see a new flourishing of scientific and social progress in this century.”
Q: What impact do you hope this essay has on the reader? How do you hope his or her thinking is changed after reading it?
A: It’s a bit of a cliché, but I want to start an open and inclusive conversation about what’s gone wrong in science, why it’s happened, and what can be done. Right now the conversation is way too balkanized, and no one seems to really want to acknowledge the larger, foundational challenges. So some people are focused on how you improve laboratory practice, and others on how you link science to better policy making, and others on how you deal with the terrible incentives that are driving a lot of science these days.
But these are just small parts of the elephant. We need to think about the whole institution and its place in our culture and society, and we need to be willing to talk about what works and what doesn’t, and to realize that the interests and power that dominate the terms of debate may not, despite their intentions, reflect the best interests of society.
If my essay empowers people to learn more and to ask questions and to join the discussion, then I’ll feel like I have done my job.
Top photo by Charlie Leight/ASU Now
More Science and technology
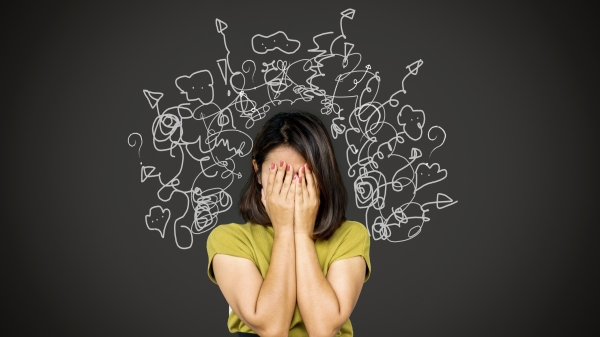
The science behind chronic stress
Stress comes in many shapes and sizes. There’s the everyday stress of preparing for a final exam or being stuck in traffic. And the more significant stress of losing a friend, family member,…
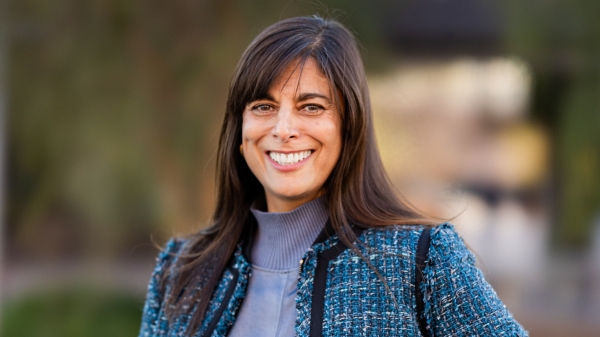
ASU planetary scientist to be inducted into the National Academy of Sciences
The National Academy of Sciences is inducting School of Earth and Space Exploration Director Meenakshi Wadhwa into the 2023 class of new members for her pioneering work in planetary sciences and…
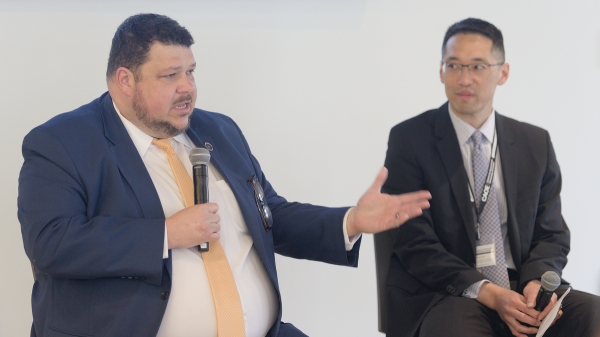
Unlocking the potential of AI for homeland security
“Can we do what we're doing now cheaper, more efficiently, more effectively?” Adam Cox, director in the Office of Strategy and Policy at the Department of Homeland Security Science and Technology…